https://yle.fi/uutiset/3-9863629
Fysiikan Nobel-palkinto myönnettiin painovoima-aaltojen löytämisestä
Fysiikan Nobel-palkinnon saavat Rainer Weiss, Barry C. Barish ja Kip S. Thorne.
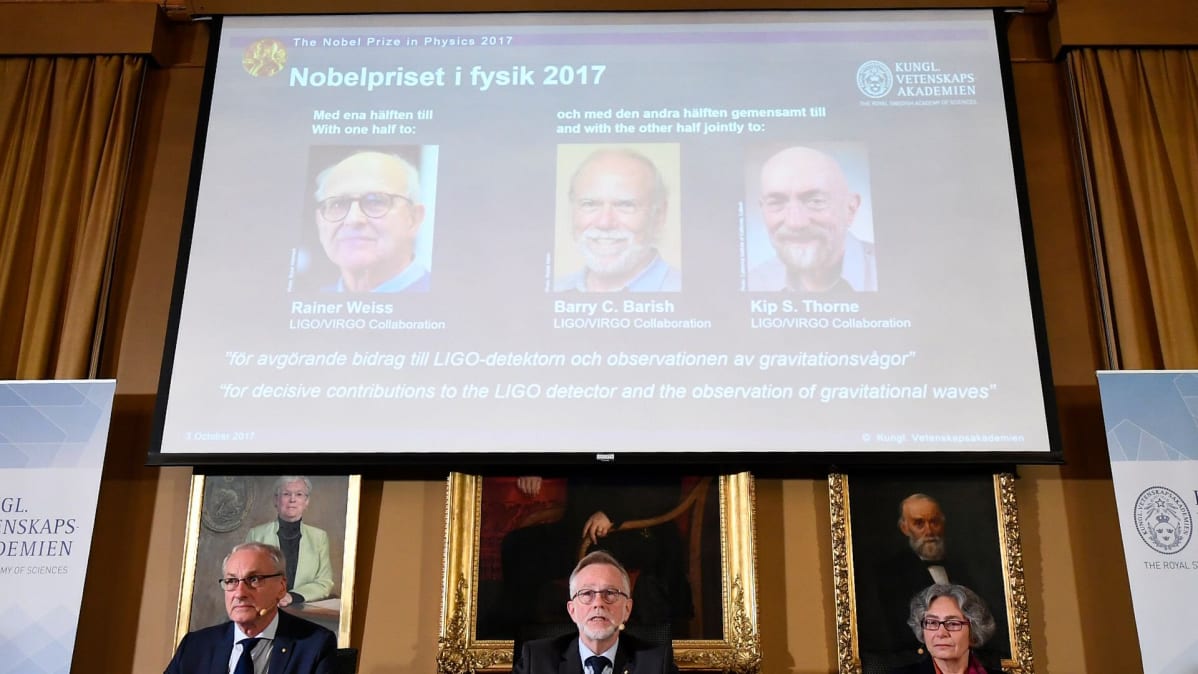
Täällä on perusteellinen esitys gravitaatioaalloista, jossa viimeisimmätkin tulokset ovat mukana.
Gravitational wave
Also found in: Dictionary, Thesaurus, Acronyms, Encyclopedia.
Related to Gravitational wave: Gravitational Radiation, Gravity waves
General relativity |
---|
![]() Gravitational waves can penetrate regions of space that electromag- netic waves cannot. They are able to allow the observation of the mer- ger of black holes and possibly other exotic objects in the distant Uni- verse. Such systems cannot be observed with more traditional means such as optical telescopes or radio telescopes, and so gravitational-wave astronomy gives new insights into the working of the Universe. In particular, gravitational waves could be of interest to cosmologists as they offer a possible way of observing the very early Universe. This is not possible with conventional astronomy, since before recombination the Universe was opaque to electromagnetic radiation. [17] Precise measurements of gravitational waves will also allow scientists to test more thoroughly the general theory of relativity. In principle, gravitational waves could exist at any frequency. However, very low frequency waves would be impossible to detect and there is no credible source for detectable waves of very high frequency. Stephen Hawking and Werner Israel list different frequency bands for gravitational waves that could plausibly be detected, ranging from 10−7 Hz up to 1011 Hz. [18] HistoryPrimordial gravitational waves are hypothesized to arise from cosmic inflation, a faster-than-light expansion just after the Big Bang (2014). [19][20][21]
.
In 1905, Henri Poincaré first suggested that in analogy to an accelera- ting electrical charge producing electromagnetic waves, accelerated masses in a relativistic field theory of gravity should produce gravita- tional waves. [22][23] When Einstein published his theory of general relativity in 1915, he was skeptical of Poincaré’s idea since the theory implied there were no ”gravitational dipoles”. Nonetheless, he still pursued the idea and based on various approximations came to the conclusion there must, in fact, be three types of gravitational wave (dubbed longitudinal-longitudinal, transverse-longitudinal, and transverse-transverse by Hermann Weyl). [23] However, the nature of Einstein’s approximations led many (including Einstein himself) to doubt the result. In 1922, Arthur Eddington showed that two of Einstein’s types of waves were artifacts of the coordinate system he used, and could be made to propagate at any speed by choosing appropriate coordinates, leading Eddington to jest that they ”propagate at the speed of thought”. [24]:72 This also cast doubt on the physicality of the third (transverse-transverse) type (which Eddington showed always propagate at the speed of light regardless of coordi- nate system). In 1936, Einstein and Nathan Rosen submitted a paper to Physical Review in which they claimed gravitational waves could not exist in the full theory of general relativity because any such solution of the field equations would have a singularity.The journal sent their manu- script to be reviewed by Howard P. Robertson, who (anonymously) re-ported that the singularities in question were simply the harmless coor- dinate singularities of the employed cylindrical coordinates. Einstein, who was unfamiliar with the concept of peer review, angrily withdrew the manuscript, never to publish in Physical Review again. Nonetheless, his assistant Leopold Infeld, who had been in contact with Robertson, convinced Einstein that the criticism was correct and the paper was rewritten with the opposite conclusion (and published elsewhere). [23][24]:79ff In 1956,Felix Pirani remedied the confusion caused by the use of vari- ous coordinate systems by rephrasing the gravitational waves in terms of the manifestly observable Riemann curvature tensor. At the time this work was mostly ignored because the community was focused on a different question: whether gravitational waves could transmit energy. This matter was settled by a thought experiment proposed by Richard Feynman during the first ”GR” conference at Chapel Hill in 1957. In short, his argument (known as the ”sticky bead argument”) notes that if one takes a stick with beads then the effect of a passing gravitational wave would be to move the beads along the stick; friction would then produce heat, implying that the passing wave had done work. Shortly after, Hermann Bondi (a former gravitational wave skeptic) published a detailed version of the ”sticky bead argument”. [23] After the Chapel Hill conference, Joseph Weber started designing and building the first gravitational wave detectors now known as Weber bars. In 1969, Weber claimed to have detected the first gravitational waves and by 1970 he was ”detecting” signals regularly from the center of Milky Way; however, the frequency of detection soon raised doubts on the validity of his observations as the implied rate of energy loss of the Milky Way would drain our galaxy of energy on a timescale much shorter than its inferred age. These doubts were strengthened when, by the mid-1970s, repeat experiments from other groups building their own Weber bars across the globe failed to find any signals, and by the late 1970s general consensus was that Weber’s results were spurious. [23] In the same period, the first indirect evidence for the existence of gravi-tational waves was discovered. In 1974, Russell Alan Hulse and Joseph Hooton Taylor, Jr. discovered the first binary pulsar (a discovery that earned them the 1993 Nobel Prize in Physics). In 1979, results were published detailing measurement of the gradual decay of the orbital period of the Hulse-Taylor pulsar, which fitted precisely with the loss of energy and angular momentum in gravitational radiation predicted by general relativity. [23] [ From prediction to reality: a history of the search for gravitational waves
|
Gravitational waves are ripples in the curvature of spacetime that pro- pagate as waves at the speed of light,generated in certain gravitational interactions that propagate outward from their source. The possibility of gravitational waves was discussed in 1893 by Oliver Heaviside using the analogy between the inverse-square law in gravitation and electrici- ty. [1] In 1905 Henri Poincaré first proposed gravitational waves (ondes gravifiques) emanating from a body and propagating at the speed of light as being required by the Lorentz transformations. [2] Predicted in 191 [3][4] by Albert Einstein on the basis of his theory of general relati- vity, [5][6] gravitational waves transport energy as gravitational radi- ation, a form of radiant energy similar to electromagnetic radiation. [7] Gravitational waves cannot exist in the Newton’s law of universal gra- vitation, since that law is predicated on the assumption that physical interactions propagate at infinite speed.
Gravitational-wave astronomy is an emerging branch of observational astronomy which aims to use gravitational waves to collect observa- tional data about sources of detectable gravitational waves such as binary star systems composed of white dwarfs, neutron stars, and black holes; and events such as supernovae, and the formation of the early universe shortly after the Big Bang.
On February 11,2016,the LIGO Scientific Collaboration and Virgo Colla- boration teams announced that they had made the first observation of gravitational waves, originating from a pair of merging black holes using the Advanced LIGO detectors. [8][9][10] Since the initial announce- ment LIGO has confirmed two more (and one potential) detections of gravitational wave events. [11][12] Besides LIGO, several other gravita- tional-wave observatories (detectors) are under construction. [13]
Introduction
In Einstein’s theory of general relativity, gravity is treated as a pheno- menon resulting from the curvature of spacetime. This curvature is caused by the presence of mass. Generally, the more mass that is contained within a given volume of space, the greater the curvature of spacetime will be at the boundary of its volume. [14] As objects with mass move around in spacetime, the curvature changes to reflect the changed locations of those objects. In certain circumstances, accele- rating objects generate changes in this curvature, which propagate outwards at the speed of light in a wave-like manner. These propagating phenomena are known as gravitational waves.
As a gravitational wave passes an observer, that observer will find spacetime distorted by the effects of strain. Distances between ob- jects increase and decrease rhythmically as the wave passes, at a fre- quency corresponding to that of the wave. This occurs despite such free objects never being subjected to an unbalanced force. The magni- tude of this effect decreases proportional to the inverse distance from the source. [15]:227 Inspiraling binary neutron stars are predicted to be a powerful source of gravitational waves as they coalesce,due to the ve- ry large acceleration of their masses as they orbit close to one another. However, due to the astronomical distances to these sources, the effects when measured on Earth are predicted to be very small, having strains of less than 1 part in 1020. Scientists have demonstrated the existence of these waves with ever more sensitive detectors. The most sensitive detector accomplished the task possessing a sensitivity measurement of about one part in 5×1022 (as of 2012) provided by the LIGO and VIRGO observatories. [16] A space based observatory, the Laser Interferometer Space Antenna, is currently under development by ESA.
Effects of passing
Gravitational waves are constantly passing Earth; however, even the strongest have a minuscule effect and their sources are generally at a great distance. For example, the waves given off by the cataclysmic final merger of GW150914 reached Earth after travelling over a billion lightyears, as a ripple in spacetime that changed the length of a 4-km LIGO arm by a ten thousandth of the width of a proton, proportionally equivalent to changing the distance to the nearest star outside the Solar System by one hair’s width. [42] This tiny effect from even extreme gravitational waves makes them undetectable on Earth by any means other than the most sophisticated detectors.
The effects of a passing gravitational wave,in an extremely exaggera- ted form, can be visualized by imagining a perfectly flat region of spacetime with a group of motionless test particles lying in a plane (e.g., the surface of a computer screen). As a gravitational wave passes through the particles along a line perpendicular to the plane of the particles (i.e. following the observer’s line of vision into the screen), the particles will follow the distortion in spacetime, oscillating in a ”cruciform” manner, as shown in the animations. The area enclosed by the test particles does not change and there is no motion along the direction of propagation.
The oscillations depicted in the animation are exaggerated for the pur- pose of discussion — in reality a gravitational wave has a very small amplitude (as formulated in linearized gravity). However, they help illus- trate the kind of oscillations associated with gravitational waves as produced, for example, by a pair of masses in a circular orbit. In this case the amplitude of the gravitational wave is constant, but its plane of polarization changes or rotates at twice the orbital rate and so the time-varying gravitational wave size (or ’periodic spacetime strain’) exhibits a variation as shown in the animation. [43] If the orbit of the masses is elliptical then the gravitational wave’s amplitude also varies with time according to Einstein’s quadrupole formula. [4]
As with other waves, there are a number of characteristics used to describe a gravitational wave:
- Amplitude: Usually denoted h, this is the size of the wave — the fraction of stretching or squeezing in the animation. The amplitude shown here is roughly h = 0.5 (or 50%). Gravitational waves passing through the Earth are many sextillion times weaker than this — h ≈ 10−20.
- Frequency: Usually denoted f, this is the frequency with which the wave oscillates (1 divided by the amount of time between two successive maximum stretches or squeezes)
- Wavelength: Usually denoted λ, this is the distance along the wave between points of maximum stretch or squeeze.
- Speed: This is the speed at which a point on the wave (for example, a point of maximum stretch or squeeze) travels. For gravitational waves with small amplitudes, this wave speed is equal to the speed of light (c).
The speed, wavelength, and frequency of a gravitational wave are related by the equation c = λ f, just like the equation for a light wave. For example, the animations shown here oscillate roughly once every two seconds. This would correspond to a frequency of 0.5 Hz, and a wave- length of about 600 000 km, or 47 times the diameter of the Earth.
In the above example, it is assumed that the wave is linearly polarized with a ”plus” polarization, written h+. Polarization of a gravitational wave is just like polarization of a light wave except that the polarizations of a gravitational wave are at 45 degrees, as opposed to 90 degrees. In particular, in a ”cross”-polarized gravitational wave, h×, the effect on the test particles would be basically the same, but rotated by 45 degrees, as shown in the second animation. Just as with light polarization, the polarizations of gravitational waves may also be expressed in terms of circularly polarized waves. Gravitational waves are polarized because of the nature of their sources.
Sources
In general terms, gravitational waves are radiated by objects whose motion involves acceleration and its change, provided that the motion is not perfectly spherically symmetric (like an expanding or contracting sphere) or rotationally symmetric (like a spinning disk or sphere). A simple example of this principle is a spinning dumbbell. If the dumbbell spins around its axis of symmetry, it will not radiate gravitational waves; if it tumbles end over end, as in the case of two planets orbiting each other, it will radiate gravitational waves. The heavier the dumbbell, and the faster it tumbles, the greater is the gravitational radiation it will give off. In an extreme case, such as when the two weights of the dumbbell are massive stars like neutron stars or black holes, orbiting each other quickly, then significant amounts of gravitational radiation would be given off.
Some more detailed examples:
- Two objects orbiting each other, as a planet would orbit the Sun, will radiate.
- A spinning non-axisymmetric planetoid — say with a large bump or dimple on the equator — will radiate.
- A supernova will radiate except in the unlikely event that the explosion is perfectly symmetric.
- An isolated non-spinning solid object moving at a constant velocity will not radiate. This can be regarded as a consequence of the principle of conservation of linear momentum.
- A spinning disk will not radiate. This can be regarded as a consequence of the principle of conservation of angular momentum. However, it will show gravitomagnetic effects.
- A spherically pulsating spherical star (non-zero monopole moment or mass, but zero quadrupole moment) will not radiate, in agreement with Birkhoff’s theorem.
More technically, the third time derivative of the quadrupole moment (or the l-th time derivative of the l-th multipole moment) of an isolated system’s stress–energy tensor must be non-zero in order for it to emit gravitational radiation. This is analogous to the changing dipole moment of charge or current that is necessary for the emission of electromagnetic radiation.
Binaries
Gravitational waves carry energy away from their sources and, in the case of orbiting bodies, this is associated with an inspiral or decrease in orbit. [45][46] Imagine for example a simple system of two masses — such as the Earth–Sun system — moving slowly compared to the speed of light in circular orbits. Assume that these two masses orbit each other in a circular orbit in the x–y plane. To a good approximation, the masses follow simple Keplerian orbits. However, such an orbit represents a changing quadrupole moment. That is, the system will give off gravitational waves.
In theory, the loss of energy through gravitational radiation could even- tually drop the Earth into the Sun. However, the total energy of the Earth orbiting the Sun (kinetic energy + gravitational potential energy) is about 1.14×1036 joules of which only 200 Watts (joules per second) is lost through gravitational radiation, leading to a decay in the orbit by about 1×10−15 meters per day or roughly the diameter of a proton. At this rate, it would take the Earth approximately 1×1013 times more than the current age of the Universe to spiral onto the Sun. This estimate overlooks the decrease in r over time, but the majority of the time the bodies are far apart and only radiating slowly, so the difference is unimportant in this example.
More generally, the rate of orbital decay can be approximated by [47]
where r is the separation between the bodies, t time, G Newton’s con- stant, c the speed of light, and m1 and m2 the masses of the bodies. This leads to an expected time to merger of [47]
For example, a pair of solar mass neutron stars in a circular orbit at a separation of 1.89×108 m (189,000 km) has an orbital period of 1,000 seconds, and an expected lifetime of 1.30×1013 seconds or about 414,000 years. Such a system could be observed by LISA if it were not too far away. A far greater number of white dwarf binaries exist with orbital periods in this range. White dwarf binaries have masses in the order of the Sun, and diameters in the order of the Earth. They cannot get much closer together than 10,000 km before they will merge and explode in a supernova which would also end the emission of gravitational waves. Until then, their gravitational radiation would be comparable to that of a neutron star binary.
When the orbit of a neutron star binary has decayed to 1.89×106 m (1890 km), its remaining lifetime is about 130,000 seconds or 36 hours. The orbital frequency will vary from 1 orbit per second at the start, to 918 orbits per second when the orbit has shrunk to 20 km at merger. The majority of gravitational radiation emitted will be at twice the orbital frequency. Just before merger, the inspiral would be observed by LIGO if such a binary were close enough. LIGO has only a few minutes to observe this merger out of a total orbital lifetime that may have been billions of years. Advanced LIGO detector should be able to detect these events up to 200 megaparsec away. Within this range of the order 40 events are expected per year. [48]
Black holes
Black hole binaries emit gravitational waves during their in-spiral, mer- ger, and ring-down phases. The largest amplitude of emission occurs during the merger phase, which can be modeled with the techniques of numerical relativity.[32][33][34] The first direct detection of gravitational waves, GW150914, came from the merger of two black holes.
Supernova
A supernova is an astronomical event that occurs during the last stellar evolutionary stages of a massive star’s life, whose dramatic and catast- rophic destruction is marked by one final titanic explosion. This ex- plosion can happen in one of many ways, but in all of them a significant proportion of the matter in the star is blown away into the surrounding space at extremely high velocities (up to 10% of the speed of light). Unless there is perfect spherical symmetry in these explosions (i.e., un- less matter is spewed out evenly in all directions), there will be gravita- tional radiation from the explosion. This is because gravitational waves are generated by a changing quadrupole moment, which can happen only when there is asymmetrical movement of masses. Since the exact mechanism by which supernovae take place is not fully understood, it is not easy to model the gravitational radiation emitted by them.
Rotating neutron stars
As noted above,a mass distribution will emit gravitational radiation only when there is spherically asymmetric motion among the masses.A spin- ning neutron star will generally emit no gravitational radiation because neutron stars are highly dense objects with a strong gravitational field that keeps them almost perfectly spherical. In some cases, however, there might be slight deformities on the surface called ”mountains”, which are bumps extending no more than 10 centimeters (4 inches) above the surface, [49] that make the spinning spherically asymmetric. This gives the star a quadrupole moment that changes with time, and it will emit gravitational waves until the deformities are smoothed out.
Inflation
Many models of the Universe postulate that there was an inflationary epoch in the early history of the Universe when space expanded by a large factor in a very short amount of time. If this expansion was not symmetric in all directions, it may have emitted gravitational radiation detectable today as a gravitational wave background.This background signal is too weak for any currently operational gravitational wave detector to observe, and it is thought it may be decades before such an observation can be made.
Properties and behaviour
Energy, momentum, and angular momentum
Water waves, sound waves, and electromagnetic waves are able to carry energy, momentum, and angular momentum and by doing so they carry those away from the source. Gravitational waves perform the same function. Thus, for example, a binary system loses angular momentum as the two orbiting objects spiral towards each other — the angular momentum is radiated away by gravitational waves.
The waves can also carry off linear momentum, a possibility that has some interesting implications for astrophysics. [50] After two super- massive black holes coalesce, emission of linear momentum can pro- duce a ”kick” with amplitude as large as 4000 km/s. This is fast enough to eject the coalesced black hole completely from its host galaxy. Even if the kick is too small to eject the black hole completely, it can remove it temporarily from the nucleus of the galaxy, after which it will oscillate about the center, eventually coming to rest. [51] A kicked black hole can also carry a star cluster with it, forming a hypecompact stellar system. [52] Or it may carry gas, allowing the recoiling black hole to appear tem- porarily as a ”naked quasar”. The quasar SDSS J092712.65+294344.0 is thought to contain a recoiling supermassive black hole. [53]
Redshifting and blueshifting
Like electromagnetic waves, gravitational waves should exhibit shif- ting of wavelength due to the relative velocities of the source and observer, but also due to distortions of space-time, such as cosmic expansion. This is the case even though gravity itself is a cause of distortions of space-time. Redshifting of gravitational waves is different from redshifting due to gravity.
Quantum gravity, wave-particle aspects, and graviton
At present, and unlike all other known forces in the universe, no ”force carrying” particle has been identified as mediating gravitational interactions.
In the framework of quantum field theory, the graviton is the name given to a hypothetical elementary particle speculated to be the force carrier that mediates gravity. However the graviton is not yet proven to exist and no reconciliation yet exists between general relativity which describes gravity, and the Standard Model which describes all other fundamental forces. (For scientific models which attempt to reconcile these, see quantum gravity).
If such a particle exists, it is expected to be massless (because the gra-vitational force appears to have unlimited range) and must be a spin-2 boson. It can be shown that any massless spin-2 field would give rise to a force indistinguishable from gravitation, because a massless spin-2 field must couple to (interact with) the stress–energy tensor in the same way that the gravitational field does; therefore if a massless spin-2 particle were ever discovered, it would be likely to be the gravi- ton without further distinction from other massless spin-2 particles. [54] Such a discovery would unite quantum theory with gravity. [55]
Significance for study of the early universe
Due to the weakness of the coupling of gravity to matter, gravitational waves experience very little absorption or scattering, even as they tra- vel over astronomical distances. In particular, gravitational waves are expected to be unaffected by the opacity of the very early universe. In these early phases, space had not yet become ”transparent,” so observations based upon light, radio waves, and other electromagnetic radiation that far back into time are limited or unavailable. Therefore, gravitational waves are expected in principle to have the potential to provide a wealth of observational data about the very early universe.
Determining direction of travel
The difficulty in directly detecting gravitational waves, means it is also difficult for a single detector to identify by itself the direction of a source. Therefore, multiple detectors are used, both to distinguish signals from other ”noise” by confirming the signal is not of earthly origin, and also to determine direction by means of triangulation. This technique uses the fact that the waves travel at the speed of light and will reach different detectors at different times depending on their source direction. Although the differences in arrival time may be just a few milliseconds, this is sufficient to identify the direction of the origin of the wave with considerable precision.
In the case of GW150914, only two detectors were operating at the time of the event, therefore, the direction is not so precisely defined and it could lie anywhere within an arc-shaped region of space rather than being identified as a single point.
Astrophysical implications
During the past century, astronomy has been revolutionized by the use of new methods for observing the universe. Astronomical observations were originally made using visible light. Galileo Galilei pioneered the use of telescopes to enhance these observations. However, visible light is only a small portion of the electromagnetic spectrum, and not all ob- jects in the distant universe shine strongly in this particular band. More useful information may be found, for example, in radio wavelengths. Using radio telescopes, astronomers have found pulsars, quasars, and other extreme objects that push the limits of our understanding of physics. Observations in the microwave band have opened our eyes to the faint imprints of the Big Bang, a discovery Stephen Hawking called the ”greatest discovery of the century, if not all time”. Similar advances in observations using gamma rays, x-rays, ultraviolet light, and infrared light have also brought new insights to astronomy. As each of these regions of the spectrum has opened, new discoveries have been made that could not have been made otherwise. Astronomers hope that the same holds true of gravitational waves. [56]
Gravitational waves have two important and unique properties. First, there is no need for any type of matter to be present nearby in order for the waves to be generated by a binary system of uncharged black holes, which would emit no electromagnetic radiation. Second, gravita- tional waves can pass through any intervening matter without being scattered significantly. Whereas light from distant stars may be blocked out by interstellar dust, for example, gravitational waves will pass through essentially unimpeded. These two features allow gravita- tional waves to carry information about astronomical phenomena heretofore never observed by humans, and as such represent a revolution in astrophysics. [57]
The sources of gravitational waves described above are in the low-fre- quency end of the gravitational-wave spectrum (10−7 to 105 Hz). An astrophysical source at the high-frequency end of the gravitational-wave spectrum (above 105 Hz and probably 1010 Hz) generates relic gravitational waves that are theorized to be faint imprints of the Big Bang like the cosmic microwave background.[58] At these high frequencies it is potentially possible that the sources may be ”man made” [18] that is, gravitational waves generated and detected in the laboratory. [59][60]
A supermassive black hole, created from the merger of the black holes at the center of two merging galaxies detected by the Hubble telescope, is theorized to have been ejected from the merger center by gravitational waves. [61][62]
… ”
Gravitaatioaallot eivät ole gravitaation selitys vaan sen hypoteettinen ominaisuus. Hypoteesin perustana on massan rinnastaminen sähkö- magneettiseen varaukseen,joka kiihtyvässä liikkeessä ollesaan säteilee fotoneja.Vastaavalla tavalla esimerkiksi toisiaan kiertävien massojen ar- vellaan säteilevän pois energiaansa gravitoneina, jotka eivät ole sähkö- magneettista säteilyä. Silti niiden nopeudeksi arvellaan hypoteettisesti valon nopeus c. Tämän perustana on mm. olettamus YHTENÄISTEO- RIASTA, sama joka on ollut Higgsin bosonin etsinnän pohjana, vaikka juuri muuta kokeellisesti vahvistettua (?) yhteyttä näiden teorioiden vä- lillä ei olekaan ollut. Tämän kanssa on kilpaillut mm. Kraussin ”sipuliver- sumiteoria”, jonka mukaa gravitaatio omonaisuuksineen olisi peräisin eri alkupaukusta kuin sähkämagnettinen voima
” keskiviikko, 3. lokakuu 2012
http://keskustelu.skepsis.fi/Message/FlatMessageIndex/355465?page=2#363834
Stephen Hawkingin uusimman kirjan ”Suuri suunnitelma” (The Grand Design, yhdessä tieteiskirjailija Mlodinovin kanssa) varsinainen pläjäys, joka on esitetty lopussa kuin ohimennen, on,että gravitaatio olisi massaenergiaan ja liike-energiaan nähden negatiivista energiaa, ja maailmankaikkeuden kokonaisenergia olisi nolla.
Potentiaalienergia ei olisikaan silloin vain laskennallinen suure, vaan ”täyttä materiaa”, jota ”kumotaan”, kun esimerkiksi kappaletta nostetaan ylöspäin gravitaatiokentässä, tai positiivista sähkövarausta siirretään niin ikään positiivisen jännitelähteen suuntaan. Ideologisesti kyseessä on jonkinlainen ”kaksoisenergetismi”, uusi fysiikan ideologia.
Kirja on kirjoitettu ennen Higgsin bosonin ”ratkaisua”, jonka Hawking ei uskonut olevan positiivinen (mutta uskoo niin nyt), ”hyvissä ajoin” uudeksi vaihtoehtoiseksi maailmankuvaksi. Kirja kannattaa multiver- sumiteoriaa ja myötäilee joissakin asioissa ”monimaailmatulkintaa”, mutta ei tarkoita aivan samaa kuin Hugh Everett ja mormonit. Kirjassa hyväksytään jollakin tavalla Everettin opettajan John Wheelerin ”ma- donreiät”, eikä siten kumota ehdottomasti (ymmärtääkseni) ”aikamat- kailua”. Tähän liittyen kirjassa esitetään useassa kohdassa, että ”valon nopeus voidaan ylittää”, esimerkiksi kirjassa väitetään, että Tsheren- kovin valonsäteily ydinvoimaloissa johtuu siitä,että ”elektronit liikku- vat vedessä valoa nopeammin” ja osa sähkökentän fotoneista ikään kuin jää niistä jälkeen. Tuosta sain ainakin minä väärän kuvan siitä, mitä haluttiin sanoa. Ainoankaan fysikaalisen olion nopeus ei ylitä valonno- peutta tyhjiössä, minkä mahdollisuuden erityinen suhteellisuusteoria kiistää.Kirjassa väitetään myös sopivalla nopeudella liikkuvan havaitsi- jan havaitsevan ”ajan kulkevan taaksepäin” jossakin versumin osassa. Tämä edellyttäisi kuitenkin, että kyseinen ”havaitsija” liikkuisi valon nopeutta suuremmalla nopeudella.
Nuo totutusta poikkeavat tulkinnat johtuvat siitä,e ttä kirjassa ei tun- nusteta ’materiaalisen olion’ (objektin) käsitettä (kuten dialektisessa ja muussa emergentissä materialismissa), joka on juuri se entiteetti joka mm. ei voi liikkua valoa nopeammin, on se sitten hiukkanen tai in- formaatiosignaali. Erilaiset muut, puhtaasti matemaattisesti ”viriteltä- vissä” olevat ”mittauskohteet” niin voivat tehdä, koska ne eivät ole fysikaalisten oloiden kokonaisuuksia. Toisaalta Tsherenkovin säteilykin voidaan tulkita johtuvan siitä, ettei todellinen olio (myöskään elektroni) voi ylittää valon nopeutta. Kiihtyvässä liikkeessä oleva sähkövaraus ylipäätään aina säteilee fotoneja. (Fotonit jossakin väliaineessa silti voivat ”jäädä jälkeen” siitä.)
Kirjassa väitetään myös, että on aivan sama ajatellaanko Maa tai Au- rinko jököttämään paikallaan ja muu versumi kiertämään sitä, vai nämä liikkumaan homogeenisessa versumissa.Noin aivan puhtaasti matema- tiikan kannalta onkin, MUTTA EI FYSIIKAN kannalta, sillä tämä edellyt- täisi äärettömiä (kierto)nopeuksia siellä ”laidoilla”,eikä erityinen suhteel- lisuusteoria, jonka nimiin tämäkin kirja muutoin vannoo, voisi pitää paik- kaansa. Kysymykseen monista ulottuvuuksista kirja suhtautuu tutun myönteisesti, ja perusteleekin, miten kolme paikkaulottuvuutta ovat erikoisasemassa voiden taata paikallisesti stabiilin avaruuden.
Hawkingin lanseeraaman ”malliriippuvaisen realismin” filosofian mal- lit eivät ole aivan samaa samaa kuin mm. dialektisen materialismin teoriat, joissa mm. käsitteet ja jopa kulloinenkin muodollinen logiikkakin voidaan määritellä kussakin erikseen. Malleilla on aina ymmärtääkseni ainakin perustavimmat käsitteet samat, mutta ne painottavat todellisuuden eri puolia ja ne yhdistetään ”rajapinnoilla”.
Niiden ero on lopultakin enemmän tiedollinen ja havainnollinen kuin ole-muksellinen. Tällainen on todellakin mallintamista enemmän kuin teori- anmuodostusta. Hawkingille ”alkuräjähdys on seurausta luonnonlaeis- ta”, joista siis perustavimmat olisivat ”ehdottoman muuttumattomia” (myös alkupaukkujen yli), joten kirjassa on syytä epäillä suhtauduttavan rivien välistä yhtä kielteisesti aitoon emergenssiin eli luonnonlakien kehitykseen kuin Kari Enqvistin yksinkertaisessa energetismissäkin.
Noihin ehdottomiin luonnonlakeihin olisi myös ”sisäänkirjoitettu” kaksi- päisen muodollisen logiikan taustaoletukset: olisi olemassa sellainen (moniulotteisen) olemisen taso, jonka ”pisteissä” mikä tahansa ominaisuus joko täysin olisi tai sellaista ei olisi lainkaan.
Ns. luonnonvakiot kuten valonnopeus tyhjiössä eivät kuitenkaan olisi perustavimpia luonnonlakeja, vaan ne olisivat ”versumivakioita”, jotka muuttuvat enemmän tai vähemmän joka ”poksahduksessa”, joiden tu- loksena olevat versumit eri vakioineen eivät ”kommunikoisi” keskenään ainakaan tavallisilla versumiensisäisillä fysiikan laeilla.
Mahdollisuuden ja todellisuuden dialektiikka teoksesta puuttuu ko- konaan, josta seuraa, kun on kysymys todennäköisyyslaeista, että jokin tämän hetken todella toteutuva ”ratkaisu” (tapaus) muka vaikuttaisi myös ”ajassa taaksepäin” (versumissa) siihen, miten sen ”rakennuspali- kat” joskus menneisyydessä ”todella ovat olleet”,eli tämä puoli EI OLISI LUONTEELTAAN PELKÄSTÄÄN TIEDOLLISTA,että tuosta asiasta vain saataisiin uusi tieto. Tämä käsitys on hienossa sopusoinnussa ”Wheele- rin madonreikien” ja ”aikamatkailun” kanssa,mutta jyrkässä ristiriidas- sa objektiivisen emergenssin kanssa. Todellisuudessa mahdollisten eli virtuaalisten hiukkasten ei tarvitse olla samanlaisia kuin aktuaalis- ten hiukkasten, vaikka ne muuttuvat sellaisiksi sopivien edellytysten vallitessa, ja vaikka niistä ehkä saadaankin fysikaalista tietoa vain niiden muuttumisten kautta aktuaalisiksi hiukkasiksi.
Ainoa mikä siinä ykskantaan kumotaan on ”älykäs luominen”:sellaista ei voi olla, eikä myöskään ”tarvita”.
En vaihda silti dialektisesta enkä muusta emergentistä materialismista tähän teoriaan: joutuessani valitsemaan kahdesta vaihtoehdosta, jossa toisessa perustavimmat luonnonlait ovat ehdottoman muuttumatto- mia, mutta MENNEISYYS voi vielä tänäänkin ”todella muuttua” kauaksikin ajassa taaksepäin (”malliriippuvaisessa realismissa”), ja toisessa taas menneisyys on täysin lukkoonlyöty (determinismin periaate) ja objektiivisesti ”se, mikä se on”, mutta LUONNONLAITKIN EVOLUOITUVAT (emergentissä kuten dialektisessa materialismissa) valitsen ilman muuta ja epäröimättä jälkimmäisen!
Nyt mahdollisesti löytymässä oleva Higgsin bosoni tai sen kaltainen (yksi tai jopa useampi) entuudestaan tuntematon hiukkanen sopii olio- teoriaan ja siten emergenttiin materialismiin hyvin. Tähän Hawkingin ideaan gravitaation ja energian/oiden keskinäissuhteista se varmaan tuo lisävaloa, kun päästään tarkempiin ja halvempiin lisätutkimuksiin energia-alueella, joka nyt tiedetään tarkoin.
Higgsin bosonihan sellaisenaan nykyisellään ei selitä gravitaatiota eikä raskasta massaa, vaan pelkästään hitaan massan (ellei sitten ole suorastaan jollakin tavalla ”positiivinen ja negatiivinen Higgsin bosoni”, joista toinen selittäisi massaa ja toinen gravitaatiota… Sitten ne ainakin olisivat aina varmasti ”tasapainossa”, kuten Hawking olettaa niiden kokonaisuutena aina olevan…
Lenin oikaisi mestarillisesti v. 1908 materialistisen filosofian pohjalta Ranskan Tiedeakatemian puheenjohtajaa Henri Poincaréta, joka oli itsenäisesti johtanut huomattavan osan erityisen suhteellisuuteorian matematiikkaa Hendrick Lorentzin muunnoksen ja Michelson-Morleyn valonnopeushavaintojen perusteella tämän teorianmuodostuksessa olevasta virheestä. Suotta jauhaa enqvistit filosofian olevan ”turhanpäiväistä”, kun eivät itse osaa sitä!
Materialismi ja empiriokritisismi:
http://keskustelu.skepsis.fi/Message/FlatMessageIndex/372461
http://www.kirjavinkit.fi/arvostelut/suuri-suunnitelma/
http://lukupiiri1.blogspot.fi/2012/04/stephen-hawking-leonard-mlodinow-suuri.html
Sata vuotta sitten Urantia -lmoitus julisti oppia absoluuttisesta gravitaatiosta ja siitä gravitaatiosta, jonka vaikutukset kokemuksen perusteella tunnemme.
Avaruuden keskuksen absoluuttinen gravitaatio on vaikuttanut aikojen alusta, mutta tunnettu ja myöhempi gravitaatio tuli olevaksi atomien sähköisen rakenteen myötä.
https://www.tapionajatukset.com/37
Väite:
Urantia -ilmoitus annettiin ihmiskunnan ulkopuolelta, mutta sitä ”tulkattiin” ihmisten asiantuntijaryhmän välityksellä, jotta se olisi helpompi ymmärtää.
Einstein saattoi olla yhtenä osallisena tuossa tulkkauksessa.
http://stefantallqvist.puheenvuoro.uusisuomi.fi/84246-a-einstein-oikoluki-urantia-kirjaa
”En malta olla mainitsematta, että eräs maassamme vieraileva fyysikko kerran kertoi minulle mitä fyysikot löysivät Princetonin sairaalassa kun he kävivät siellä heti Einsteinin kuoleman jälkeen. Einsteinin kuolinvuoteelta löytyi Urantia-kirja, ”The Urantia Book”!”